Part XXI: Paul Nurse, 2001 Prize in Physiology or Medicine
Joseph Luna
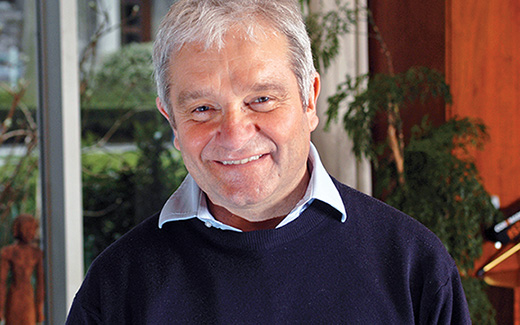
All cells, in the end, are copies of copies. But unlike the loss of quality in the Xerox sense of making a copy, a cell needs to be perfect. It must faithfully and exactly duplicate its genetic information, gather extra membranes, energy and microtubules, and then begin a dramatic line dance to separate its two genomes during mitosis. This entire process—known as the cell cycle—ensures the timely and correct reproduction of cells that is crucial for the growth of any organism.
But from the time of Virchow’s famous 1850s epigram that all cells come from cells (Omnis cellula e cellula) through the birth of molecular biology in the 1950s, all a biologist could do was watch this central process of development. The awesome molecular logistics that made the cell cycle so precise and ordered were a mystery. Who, from a molecular perspective, was in charge? How did a cell know when to execute a particular phase of the cell cycle? These questions weren’t just idle puzzles, for by this time it already been suggested, that many cell proliferative diseases such as cancer might be manifestations of cell cycles gone horribly wrong.
In 1974, a young post-doc named Paul Nurse set out to explore the cell cycle in fission yeast (Schizosaccharomyces pombe). Fresh from earning his PhD, Nurse spent half a year learning the genetics of Sz. Pombe with Urs Leopold before joining the laboratory of Murdoch Mitchinson, a pioneer of fission yeast genetics in Scotland. Nurse was inspired by the work of Leland Hartwell, who devised a way to isolate mutants of budding yeast (Saccharomyces cerevisiae) that were stuck in their progression through the cell cycle. Because such mutations were lethal, Hartwell relied on a quirk of yeast genetics that permitted temperature sensitive mutations: the yeast divided normally at lower permissive temperatures, but at higher temperatures, mutations would become apparent, and were usually lethal. Through the painstaking work of taking time-lapse photographs of many yeast mutants, Hartwell identified dozens of cell division cycle (cdc) mutants, each displaying a distinct problem in their cell cycle.
Nurse decided to apply a similar approach to rod-shaped fission yeast, which on paper, seemed tailor-made for such studies. Unlike budding yeast, fission yeast grows at a fixed diameter, and cells partition automatically once lengthened to roughly double their size. Nurse figured that cell cycle mutants would be unable to separate, and so should yield lengthened rods that were whole multiples of a single cell. Reasoning that such mutant cells were heavier, Nurse had the bright idea of trying to isolate them with a centrifuge instead of laboriously screening with the microscope.
The problem was that it failed spectacularly. The proposed mutants showed up but weren’t faulty for a specific gene in their cell cycles; Nurse’s new procedure actually yielded less cdc mutants than random visual screening. Instead, Nurse noticed something totally unexpected: microcolonies composed of small cells, much tinier than the normal rod shaped yeast. It was yet another annoying confirmation that his scheme to enrich for larger cells had failed. In frustration, in desperation, in defiance, in blind hope, or some combination thereof, he next had a truly wild idea. What if the small cells were small because they were dividing too quickly? In one fell swoop, Nurse reimagined these small cells as deficient in a step of the cell cycle that normally controls the overall rate of cell reproduction.
He named this first mutant wee (a Scottish word for small) and by chance it happened to be temperature-sensitive. By shifting temperatures of wee1 mutant cells at various stages of the cell cycle, Nurse worked out that wee1 controlled entry into mitosis–a surprising finding, since most thought that the cycle was controlled at a much earlier step known as G1. Bolstered by these results, Nurse set out to find other cell cycle genes mutated in wee cells.
More frustration ensued: he isolated 47 wee mutants that were all the same as wee1. But on the 48th try, Nurse isolated a new mutant, and named it wee2. Unlike wee1 cells, where the loss of a gene allowed the cells to divide more rapidly, wee2 cells accomplished the same rapid division due to an activating gene that was now hyperactive. Such a gain-of-function mutation could be expected to be rare. Fine genetic mapping soon gave a real surprise in that wee2 corresponded to one of Hartwell’s budding yeast mutants called cdc2. Here now were two very different mutants of the same gene in related organisms: Hartwell’s loss-of-function cdc2 mutant that halted the cell cycle, and Nurse’s wee2 mutant of the same gene that put the cell cycle in overdrive. Such odd behavior suggested that cdc2 was a critical regulator of the entire process. Formal confirmation that wee2 and cdc2 were the same came from complementation studies, where the mutant was rescued by re-introducing the normal gene. In the pre-PCR (polymerase chain reaction) era, this was laborious and heroic work. But once confirmed, it set the stage for key experiments aimed at determining whether this whole exercise was unique to yeast, or if it applied to all eukaryotic cells.
Now with his own lab, Nurse and his post-doc Melanie Lee sought to determine if human cells had a version of cdc2. The approach they eventually settled on can only be described as crazy: rescue the yeast cdc2 defect by re-introducing the human version, if there was one. With an estimated 1.5 billion years of evolutionary divergence between humans and yeast, this was a highly unlikely proposition. But if true, it would strongly suggest that cdc2 was so critical that its function had been preserved for billions of years. The risky gamble paid off. Lee and Nurse succeeded in getting broken yeast cells to divide by using the human version of cdc2, but the conclusive eureka moment came one day in front of a computer screen. After ruling out possible confounding explanations for the rescue experiment, Lee and Nurse sequenced the human cdc2 and compared it to the yeast version. Their protein sequences were 60% identical, and differed in length by one amino acid. Such evidence confirmed that these distant cousins were functionally equivalent despite a huge evolutionary distance. Countless cell divisions in innumerable organisms could be said to rely on a cdc2 molecule.