Part XV: Christian de Duve, 1974 Prize in Physiology or Medicine.
By Joseph Luna
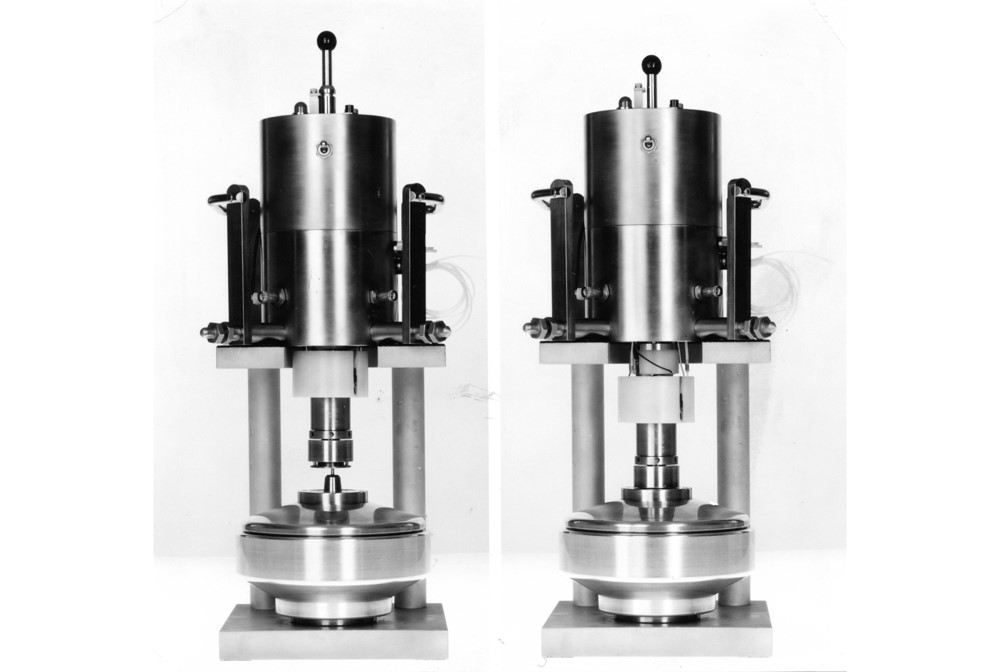
In his two-volume book A Guided Tour of the Living Cell, Christian de Duve vividly describes a most hostile setting, where “everywhere we look are scenes of destruction: maimed molecules of various kinds, shapeless debris, half-recognizable pieces of bacteria and viruses, fragments of mitochondria, membrane whorls, damaged ribosomes, all in the process of dissolving before our very eyes.” Such is the introduction to an organelle called the lysosome that only de Duve as its discoverer could give.
Where mitochondria produce energy and ribosomes produce protein, lysosomes function as a sort of digestive system for a cell: they are equal parts stomach, trash compactor, and recycling center. As bags filled with destructive enzymes, lysosomes perform the critical and often unrewarding job of waste disposal. But the story of how lysosomes were discovered was anything but unrewarding. Like any good scientific caper, it starts with a serendipitous and chance observation made under unlikely circumstances. And for the bench scientist, these circumstances were of the most frustrating variety: they all center on a positive control that never worked.
In the early 1950s, de Duve was a new faculty member at the Catholic University of Louvain in his native Belgium, and had set up his lab to tackle the mechanism of insulin on the liver. With the exception of glycolysis and the tricarboxylic acid (citric acid) cycle, metabolism was still largely uncharted territory, and one of the key questions centered on how liver cells responded to insulin to lower blood sugar. Biochemists had a hint that the first thing an insulin treated liver cell did to incoming glucose was to add a phosphate group, but this fragile phosphate group could be removed by a newly-described enzyme, later termed glucose-6-phosphatase, that generally made studying insulin action in ground-up liver tissue difficult. De Duve set out to purify and characterize this new enzyme.
After trying all the usual biochemical techniques to separate glucose-6-phosphatase from the other non-specific acid phosphatase found in the liver, de Duve hit an impasse: he couldn’t get glucose-6-phosphatase back into solution. Standard practice was to lower the pH to get an enzyme to fall out of solution, discard all the soluble stuff, and then try to get the enzyme back into solution by raising the pH. It was great on paper, except that it never worked. Luckily, de Duve was prepared.
Prior to taking up his post in Belgium, de Duve paid a visit to Albert Claude, a fellow Belgian and pioneering cell biologist then at the Rockefeller Institute. Claude had shown de Duve that proteins bound to larger structures tended to clump and stay clumped together at low pH. Thus, the most promising way to isolate glucose-6-phosphatase, if it was indeed bound to a larger structure, was to use the centrifuge of cell biologists instead of the acids used by biochemists.
It was a laborious leap of faith, requiring new reagents and expensive instruments that de Duve and his team had never used before. As every scientist wading into new technical territory knows, it was essential to make sure the new technique was working: a positive control was paramount. For fractionation studies of the liver, it just so happened that the non-specific acid phosphatase was the ideal enzyme to serve as a positive control. The basic idea was to break open liver cells and measure the activity of the enzyme in this “homogenate.” Set that value to 100 percent. It stands to reason that upon fractionating and measuring the enzymatic activity in each fraction, the sum of all fractions, like a molecular balance sheet, should equal 100 percent. For the glucose-6-phospatase enzyme, this fractionation scheme worked perfectly, and de Duve found that this enzyme was indeed associated with a larger structure in the “microsomal” fraction (later found to be the endoplasmic reticulum). But for the acid phosphatase, the sure bet that would’ve given everyone confidence that they were performing the fractionation correctly, results were all over the place. To start, the initial cell disruption techniques used by the Rockefeller group caused a 90% loss of acid phosphatase activity compared to the conditions that de Duve was used to. It was as if this enzyme had disappeared. Fractionating this homogenate unmasked some of the enzymatic activity such that the balance sheet yielded a 200% increase. Even stranger, if the homogenate was neglected and stored in the fridge for 5 days, suddenly it had 100% activity that fractionated and behaved as expected.
Any normal scientist would’ve thrown up his or her hands at such bizarre results. And since this acid phosphatase enzyme was a control that had nothing to do with the original research question on insulin in liver cells, an assistant professor would’ve been justified in dropping acid phosphatase for the sake of the lab’s stated goal. Lab notebooks, then as now are filled with such false starts and seemingly uninterpretable results.
But de Duve didn’t drop it. He became hooked on solving the riddle of the vanishing enzyme and in short order, he and his team abandoned insulin to work on this puzzle. Unlike the harsh biochemical treatment de Duve was accustomed to, the gentler isotonic buffers used to fractionate preserved cell structures, so perhaps acid phosphatase was tucked away in an inaccessible compartment that after five days in the fridge, had broken down enough to freely release the active enzyme. This hypothesis, termed “structure-linked latency” guided de Duve and his lab to determine that acid phosphatase was enclosed in a sac-like particle. Hunting these particles down with the centrifuge, he found that they settled between the mitochondrial and microsomal fraction. Soon, all manner of digestive enzymes were found in this new fraction, called the lysosomal fraction, and behaved like acid phosphatase. Electron micrograph pictures of lysosomes followed shortly thereafter. And to think it all came from failed control experiments!