The simple but bold 68th Street entrance to the Rockefeller campus was erected in honor of the man who in many ways embodies the scientific and social spirit of the institute. The inscription on one of the piers guarding the entrance reads,
and is a homage to the seminal work done over many decades by Avery and his colleagues within these gates. “The Professor” or simply “Fess” Avery, as his friends and colleagues fondly call him, had a lot in common with the institute he called home for most of his professional life. Quoting from Rene J. Dubos’ fascinating book The Professor, The Institute, and DNA (which I have used as the primary reference for this article),
“Avery and the Institute were respectively the human and institutional expressions of the same scientific attitudes. They both emerged and developed in the atmosphere of expectancy generated by a few triumphs of scientific medicine at the end of the nineteenth century; both followed an intellectual course that led them from the study of specific diseases to large problems of theoretical biology; both became part of a culture in which laboratory scientists were regarded as members of a kind of priesthood, willing to accept social constraints for the sake of intellectual privileges.”
Therefore, learning about Avery’s story allows us to delve into the fascinating history of the Rockefeller Institute and the people who shaped it. Furthermore, the 80th anniversary of Avery’s groundbreaking 1944 paper that identified DNA as the molecular basis of heredity is the perfect opportunity to recall the amazing discoveries that originated at Rockefeller and went on to shape the course of modern biomedical research.
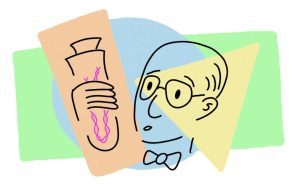
Bridging medical and laboratory sciences
Over centuries, medical science has undergone many paradigm shifts. One such noteworthy transformation occurred during the latter half of the 19th century. Due to limitations in technology, early medical science was largely empirical–the observations regarding transmission and pathologies of diseases were recorded, but the underlying mechanism remained poorly understood. As a result, the medical sciences, which dealt with patient care and treatment, were considered largely disparate from the laboratory sciences, which dealt with the chemical properties of biomolecules. However, by the late 19th century, when infectious diseases were the leading cause of death in humans, scientists like Louis Pasteur, Robert Koch, and others were beginning to demonstrate that bacteria and other microorganisms are the underlying causes of these infectious diseases. This had immediate practical consequences in the prevention and control of these ailments, and, for perhaps the first time, it was evident that progress in medicine could be achieved by laboratory investigations that did not directly involve patient care. This realization began to bridge the divide between the laboratory and medical sciences.
Medical research comes to the U.S.
Across Europe, institutions dedicated to the advancement of medicine through the study of fundamental mechanisms of pathology began emerging, such as the Pasteur Institute in Paris and the Koch Institute in Berlin. Although the prospects for medical research in the United States looked bleak initially, it soon began to change around the turn of the 20th century. During the late 19th century, it was becoming increasingly common for young American physicians to spend a few months or years in Europe, familiarizing themselves with the new kind of medical science flourishing across its medical centers. Upon returning home, they brought with them the culture of research-driven medicine that they were eager to emulate. Around the same time, wealthy philanthropists were beginning to shift the emphasis from traditional individualized charities to donating towards programs for social improvement. Together, these two factors catalyzed the creation of institutions where the new model of research-based medical science could be implemented. In addition to places like the Johns Hopkins Institute, one of the main beneficiaries of this new social phenomenon was the Rockefeller Institute, funded by the immense fortune of the oil baron John D. Rockefeller, and created with the ambitious and rather broad vision of promoting any scientific investigation with bearing on health and disease.
Oswald Avery enters the scene
The story of Oswald Avery reflected this larger trend in society. He completed his medical degree at The College of Physicians and Surgeons at Columbia University in 1904. By 1907, he had transitioned from a clinical to a more laboratory-focused role, which was fitting within the increasing research consciousness of medical New York. His first research position was as the assistant director at the Hoagland Laboratory in Brooklyn, which was amongst the first wave of privately endowed medical research laboratories in the United States. Avery spent 6 years at the Hoagland Laboratory studying and researching bacteriology, where his director Benjamin White, a Yale-educated physiological chemist, indoctrinated him with the chemical mode of thinking about biological problems—an approach that greatly inspired Avery’s future research.
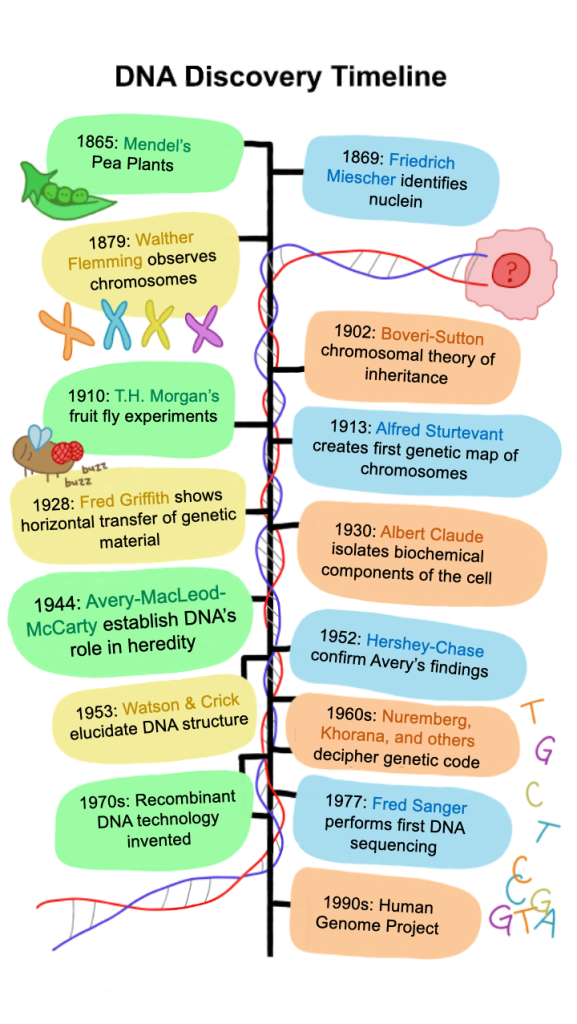
During his time at the Hoagland laboratory, Avery published nine papers related to tuberculosis, vaccinations, and secondary infections, catching the attention of Dr. Rufus Cole, the then-director of the Rockefeller Institute Hospital. In 1913, Cole recruited Avery to the pneumonia research program at the hospital, where in a few years, Avery was quickly promoted to the highest rank of a full Member. During his early years at Rockefeller, Avery’s research style also changed markedly from the more methodical but perhaps unimaginative experiments he did during his time at the Hoagland Laboratories to a more creative but still equally thorough approach that would come to characterize much of his later work. This shift in approach was likely due to the carefully cultivated intellectual culture at the Rockefeller Institute that encouraged bold and imaginative scientific pursuits largely unencumbered with funding or logistical concerns, which fit well with Avery’s innate scientific temperament. Thus began Avery’s decades-long scientific journey toward fundamental problems in biological chemistry that eventually led to the landmark 1944 paper for which he is best remembered.
The DNA revolution
In the first half of the 20th century, driven by the revolutions in genetics and molecular biology, life sciences underwent a radical transformation from a largely descriptive science to an information science. The emerging interpretation was one where the information is stored in the genetic blueprint carried by each organism, and life processes are an outcome of “reading out” this blueprint (see Figure 1). This revolution in biology was heralded by Darwin’s theory of evolution and Mendel’s genetics experiments, which laid the framework for thinking about heredity and information transfer. However, neither of their theories talked about the physicochemical mechanisms underlying their observations. The first inklings of the molecular substrate of heredity can be traced back to Walther Flemming who observed structures in the nucleus of cells that were stained by various dyes and thus named them “colored bodies” or chromosomes. Later Boveri and Sutton as well as T.H. Morgan observed how these chromosomes are transmitted during cell divisions and between generations, and noted that they followed all the rules outlined for the heredity “factors” proposed by Mendel’s theory. In parallel, other scientists pioneered techniques to isolate different chemical components of cells based on differences in their chemical properties, leading to the isolation and characterization of several important biomolecules such as RNA, DNA, lipids, and proteins. Due to the rich biochemical diversity in the composition and properties of proteins, the scientific community was quick to nominate them as the best molecular candidates for encoding genetic information. Ultimately, this protein-based heredity dogma in the field of genetics was challenged from unlikely quarters–the seemingly disconnected field of bacterial immunology.
The key experiments that established DNA’s role in heredity
During the period of 1920s-30s that came to be known as “The Golden Era of Immunology at The Rockefeller Institute,” Avery and his contemporaries made key discoveries regarding bacterial metabolism, the chemical basis of virulence and immunity, and the heritable variability in these properties between different subclasses of pneumococci. While this was happening in New York, across the Atlantic an English scientist named Fred Griffith performed his now iconic experiment (see Figure 2) where he observed that when a mixture of avirulent R strain and heat-killed virulent S strain pneumococcal bacteria is injected in mice, there is a transfer of these heritable virulence-conferring chemical properties from the latter to the former. These experiments sent shockwaves across the international immunology community and were also widely discussed in Avery’s department at the Rockefeller Institute. Avery’s group meticulously replicated these results and even extended them to demonstrate that this transformation between different bacterial cells can occur in vitro. They further showed that this in vitro transformation could be brought about not just by whole heat-killed S cells but also with a soluble fraction produced by dissolving the S cells in sodium deoxycholate (an ionic detergent) and filtering the cellular debris; the active material could be precipitated from the filtrate with alcohol and was described as “a thick syrupy precipitate” that was “fairly stable.”
Characteristic of his thorough and disciplined approach towards science, Avery spent many subsequent years trying to establish the chemical identity of this viscous precipitate that they had named the “transforming principle,” perhaps because he had sensed its broader significance as the potential molecular candidate for heredity that the field of genetics was desperately hunting. In this endeavor, Avery collaborated with many of the newer members in the department amongst which two key figures were Colin MacLeod, who helped optimize the technique for extracting highly pure samples of this transformation substance, and later Maclyn McCarty who performed many chemical tests to help establish the identity of this purified transformation substance. Some of the key chemical tests performed showed that the transforming principle was stable to the action of a myriad of proteases and ribonucleases whilst only responding to enzymes previously shown to attack deoxyribonucleic acids, showing that its elemental phosphorus-nitrogen ratios closely resembled that of DNA, and roughly estimating the molecular weight of the substance being consistent with it being a long polymer. Eventually, these results were compiled in the now classic paper by Avery, MacLeod, and McCarty submitted to the Journal of Experimental Medicine in November 1943 and published in 1944. The somewhat unflashy name of the article “Studies on the Chemical Nature of the Substance Inducing Transformation of Pneumococcal Types: Induction of Transformation by a Desoxyribonucleic Acid Fraction Isolated from Pneumococcus Type III” contrasts the extraordinary findings detailed within, which for the first time implicated that DNA could be the molecular identity of the information blueprint prevalent in all life forms. While Avery himself was conservative about making such broad claims, the scientific community understood his discovery as pivotal.
Since this discovery overturned the long-held protein-based heredity dogma, there was great resistance from the scientific community both within and outside the institute to accept these findings. Amongst the many criticisms leveled against these findings was that despite the meticulous efforts at purification, Avery’s DNA sample was somehow contaminated by small amounts of some protein which was the true “transforming principle”, or that there was some nucleoprotein so tightly associated with the DNA that it became chemically inseparable. Avery himself had anticipated this backlash and therefore had sent the manuscript of his paper for critical review to many of his friends and associates before submitting it for publication. Even after its publication, Avery was not one to broadcast his findings as a turning point in science, and characteristic of his personality, his response to the criticisms was to begin planning further experiments that could vindicate his results. Subsequently, many experiments done at Rockefeller and elsewhere provided additional evidence for DNA’s role in the transmission of hereditary characteristics. Avery’s introverted nature meant that his reactions to the happenings were rarely expressed in public, but his mood of excitement tempered with caution was evident in a letter he wrote to his brother Roy in 1943, where he recognized the broad implications of his findings, but advised him not to “shout it around” because “It’s hazardous to go off half-cocked – and embarrassing to retract it later…”.
It is hard to estimate when this tide of opinion slowly shifted, but some 8 years later, when the Hershey-Chase experiment at the Cold Spring Harbor laboratory beautifully corroborated Avery-MacLeod-McCarty’s findings, the last remaining skeptics were converted. DNA was thus incorporated into the standard genetic theory, and, in 1953, the identification of the structure of DNA by Watson and Crick with data from Rosalind Franklin ushered in an age of biology united under a few fundamental principles. This led to the coming of age of molecular biology, where the information transfer in biology was established in concrete molecular terms, compiled in what is now known as the central dogma. In turn, this paved the way for the Genomics Era, setting the stage for a time where DNA/RNA sequencing has now become a routine part of many biological experiments.
The staggering implications of these discoveries have touched every area of biology, and the ability to use a molecular language to describe essential life processes has far-reaching consequences for all of medical science. In a sense, all modern chemical genetics owes its roots to the studies in bacterial heritability done by Avery and colleagues here at the Rockefeller Institute, and it surely gives us immense pride to be a part of this great scientific legacy.