Part XXII: Roderick MacKinnon, 2003 Prize in Chemistry
Joseph Luna
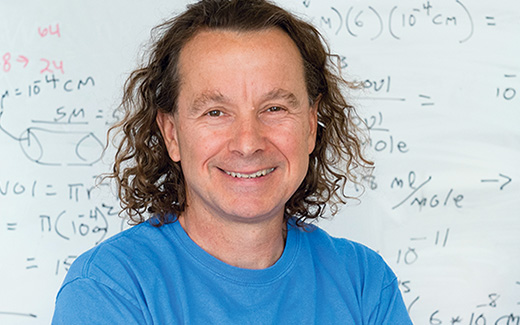
In the early 1950s, two English physiologists named Alan Hodgkin and Andrew Huxley wrote a five-part magnum opus of papers formally describing the electrochemical basis of action potentials, those short lasting impulses that travel along nerve cells. Starting with electrophysiological measurements of squid giant axons, they formulated a precise mathematical model of how action potentials arise and propagate based on the movement of small charged atoms called ions, across a cell membrane. Hodgkin and Huxley made their way to Stockholm in 1963 for this work, having achieved a true breakthrough in neuroscience. Yet such a complete synthesis was more of a molecular starting point founded on a key assumption: the Hodgkin-Huxley model critically relied on the idea that the cell membrane underwent transient changes in ion permeability. In other words, the cell membrane possessed a highly optimized border control system that would permit some ions in (or out) at one specific time and place, but not at others. How such a system actually worked at the molecular level could only be guessed at. For their part, Hodgkin and Huxley dryly wrote that the “details of the mechanism will probably not be settled for some time.” Their assumptions turned into predictions—the richest of guides for future scientists, among them Roderick MacKinnon.
One vital element of the Hodgkin-Huxley model that captured MacKinnon’s fascination centered on potassium ions (K+) and the heroic feat they needed to pull off to escape the cell. With a radius of 1.38 Ångströms, these water-loving ions manage to cross a cell membrane that resembles a great wall of grease, over 40 Ångströms thick. This would roughly translate into a barrier eight stories tall for a human sized potassium ion—scalable perhaps by Superman, were the building not made of solid Krypton. K+ ions can’t manage such an exploit alone. To get around this, Hodgkin and Huxley postulated the existence of a channel that would ferret K+ ions out of the cell. Despite the idleness implied by the name, the channel they predicted was no ordinary hallway for K+ ions. For the Hodgkin-Huxley model to work, this channel needed to be a complex machine capable of differentiating K+ ions from among scores of other (often smaller) ions, and it also needed to open and close at precise moments. In other words, it was a very selective gate.
For MacKinnon, this presented a tantalizing puzzle to determine the molecular basis of ion selectivity. How did the channel conduct potassium ions, but not others, such as physically smaller sodium (Na+) ions? After undergraduate thesis research in Chris Miller’s laboratory at Brandeis University, MacKinnon took a slight detour to go to medical school, before finding himself back in the Miller lab, thirty years old and feeling behind as a scientist, for post-doctoral work. He quickly caught up, and found himself amidst exciting times for ion channel research in the late 1980s. As a postdoc, MacKinnon worked out the mechanism of how a scorpion venom toxin blocked K+ channels in skeletal muscle (it plugged the pore). The first K+ channel called Shaker was cloned from fruit flies around the same time. Performing a “let’s see what happens” experiment, MacKinnon determined that the scorpion toxin also blocked the Shaker channel. This was fortuitous, since it meant that the specific amino acids that interacted with the toxin could be mapped to help define the pore of the channel. It was a solid first step that harnessed the power of molecular biology to explain potassium selectivity. Over the next few years, MacKinnon with his newly established lab at Harvard, determined which amino acids were essential for potassium conductance, and in broad strokes, worked out what the channel ought to look like. They imagined a tetramer of protein subunits encircling a central pore that could open and close, and where each subunit contributed a loop of amino acids whose job it was to discriminate K+ ions. And yet, despite a wealth of biophysical and biochemical data, a satisfying explanation of how the channel conducted potassium much better than smaller sodium ions remained elusive. MacKinnon sought to “see” an ion channel.
So enthralling a problem was ion selectivity that MacKinnon took up the idiosyncratic and often temperamental branch of biophysics known as X-ray crystallography to solve the atomic structure of a potassium channel. It was a bold move, and one that a place like Rockefeller, then under Torsten Weisel’s guidance, was eager to support. Now in a new field and in a new place, MacKinnon and his team got to work on solving the structure of a membrane protein. That prospect alone would make most crystallographers shudder. But a more pressing problem was making enough protein to crystallize. Because K+ channels conduct so rapidly, not many are required for normal cellular function, which means that they tend not to be very abundant and thus difficult to purify to high levels. Fortunately, by the mid-1990s enough sequencing evidence had accumulated to suggest that certain species of bacteria might encode potassium channels that could be readily grown to high levels. Moreover, many of the key sequence features were shared between bacteria and the fruit fly Shaker channel. Within two years of arriving at Rockefeller, MacKinnon and his team reported in 1998 the structure of a K+ channel called KcsA from the bacterium Streptomyces lividans. The structure at long last helped reveal the mechanism of potassium selectivity in the pore: the amino acids that lined the pore precisely mimic the way that water molecules surround, that is hydrate, a potassium ion. It is as if the channel, when open, is invisible to potassium. Sodium, however, has a harder time. Because the fit for sodium in the pore isn’t perfect, it attempts to drag in a water molecule to remain properly hydrated and the channel simply will not permit the extra baggage.
This was but a start. The structure of the potassium channel opened the door to understand a multitude of fascinating molecular features of these machines, from further studies of the pore, to details of gating to channels of other organisms. The exciting portraits of these proteins verified many key predictions of the Hodgkin-Huxley model. In a gracious gesture, MacKinnon wrote to an ailing Sir Alan Hodgkin, telling him of the news, in atomic detail.