Part XIX: Günter Blobel, 1999 Prize in Physiology or Medicine
By Joseph Luna
Let’s start with a fantastical scene: picture a band of Neolithic humans in a hot air balloon overlooking modern New York City. What would they see and experience? Lacking a vocabulary and a mental model of twenty-first century life, our ancient friends would be awestruck at seeing miniscule specks and strangely ordered structures, lines and squares, in green and gray. Perhaps the occasional yellow rectangle from which specks would enter and exit would catch their attention. Or they might ponder a box with flashing lights, speeding its way across a grid. It’s near impossible to imagine being in their shoes, but it’s easy to envision the excitement as they try to describe and make sense of what they saw.
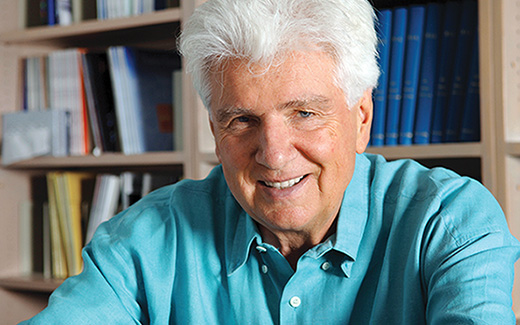
This totally novel experience wasn’t far off from what early cell biologists encountered, as they used the electron microscope (EM) as a sort of hot-air balloon to discover the cities inside cells. By the mid-1960s, they had plotted the geography of all sorts of cellular worlds, had given names to energy-making blobs and recycling vesicles, and with the help of radioactive amino acid labeling, had a basic sense of where proteins were made and where they ended up. But big questions remained such as how did a protein know where it needed to go? For a discipline built on EM observations from high above, this was a challenging question to answer, but it captivated a young German post-doc enough to dream as if he landed his hot air balloon and walked among molecules, where the view was much clearer.
Günter Blobel arrived in George Palade’s laboratory in 1967, shortly after completing his PhD at the University of Wisconsin at Madison. He joined a dynamic group of researchers who had stumbled upon an odd observation concerning the protein factories of the cell, its ribosomes: proteins destined to remain inside the cell were often made from a pool of freely cytoplasmic ribosomes, whereas proteins meant to be exported from the cell quickly associated with ribosomes attached to the endoplasmic reticulum (ER). How a new protein made this decision to stay in the cytoplasm or go to the ER was a mystery.
Within a few years, and overwhelmingly without much evidence, Blobel and a colleague (and Rockefeller University alum) named David Sabatini formulated what became known as “the signal hypothesis” that might explain how proteins got sorted to their proper locations. It represented a truly imaginative and startlingly precise leap, as if one could envision a five digit postal code and a stamp authentication system simply by watching mail trucks from space. Blobel and Sabatini proposed that ER destined proteins contained a special stretch of amino acids that acted like a signal that became apparent the moment the protein was being made at a ribosome. This signal sequence, located at the head of a protein, would be recognized by a factor (or factors) that would, in turn guide the synthesizing ribosome to the ER, where the protein in question could finish being born as it translocated across the ER membrane. Once properly sorted into the ER, the signal sequence was no longer needed and could be removed by an enzyme, even while the protein was still being made. Once finished, the protein could then go and do its job.
For many, this all sounded needlessly baroque. One attractive alternative was to consider different types of ribosomes, where each type was responsible for ferrying a nascent protein to a particular location. Another idea postulated that the mRNAs encoding proteins somehow got to the correct place before undergoing translation from any nearby ribosome. The signal hypothesis was one of many possible models, and a far-fetched one at that. But it made very precise predictions that could be tested, the first of which was the existence of a transient signal sequence.
Myeloma cells provided the first toe-hold for testing the signal hypotheses, since they secreted lots of IgG antibody light chains that could be readily detected. Using cell-free translation systems, based on these cells, other laboratories had observed slightly heftier IgG molecules than those secreted from intact cells, suggesting that a larger precursor was made and pruned to a final, smaller form. Yet, worries of an in vitro artifact abound. Blobel first repeated this experiment, and once confirmed, tinkered with his cell free system to uncover the order of events. Using detergent, he separated ribosomes from bits of ER (called microsomes) and added a drug that blocked new IgG production. He then let the ribosomes that had already started making an IgG to finish, keeping track of what they produced and when. Early in the experiment, only the smaller form emerged, which made sense if these ribosomes had already been at the ER and were nearly finished making IgG when Blobel had isolated them. But later in the experiment, a mixture of larger and smaller forms showed up: ribosomes that had just started making IgG indeed made a larger version. But lacking sufficient ER targeting, the signal sequence wasn’t pruned efficiently. Blobel had glimpsed a totally new feature in the early lives of proteins.
This was just the start. Over the ensuing years, Blobel and his team devised ways of recapitulating numerous aspects of protein targeting in the cell, from isolating the complex that ferried a signal sequence bearing protein to the ER (the aptly named “signal recognition particle”) to later confirming and characterizing the protein channel at the ER (the translocon) that nascent proteins traversed for proper processing. In part because of Blobel’s efforts, the hot air balloon view gave way to detailed explorations from the ground. A dream, as all good hypotheses are, turned out to be true.